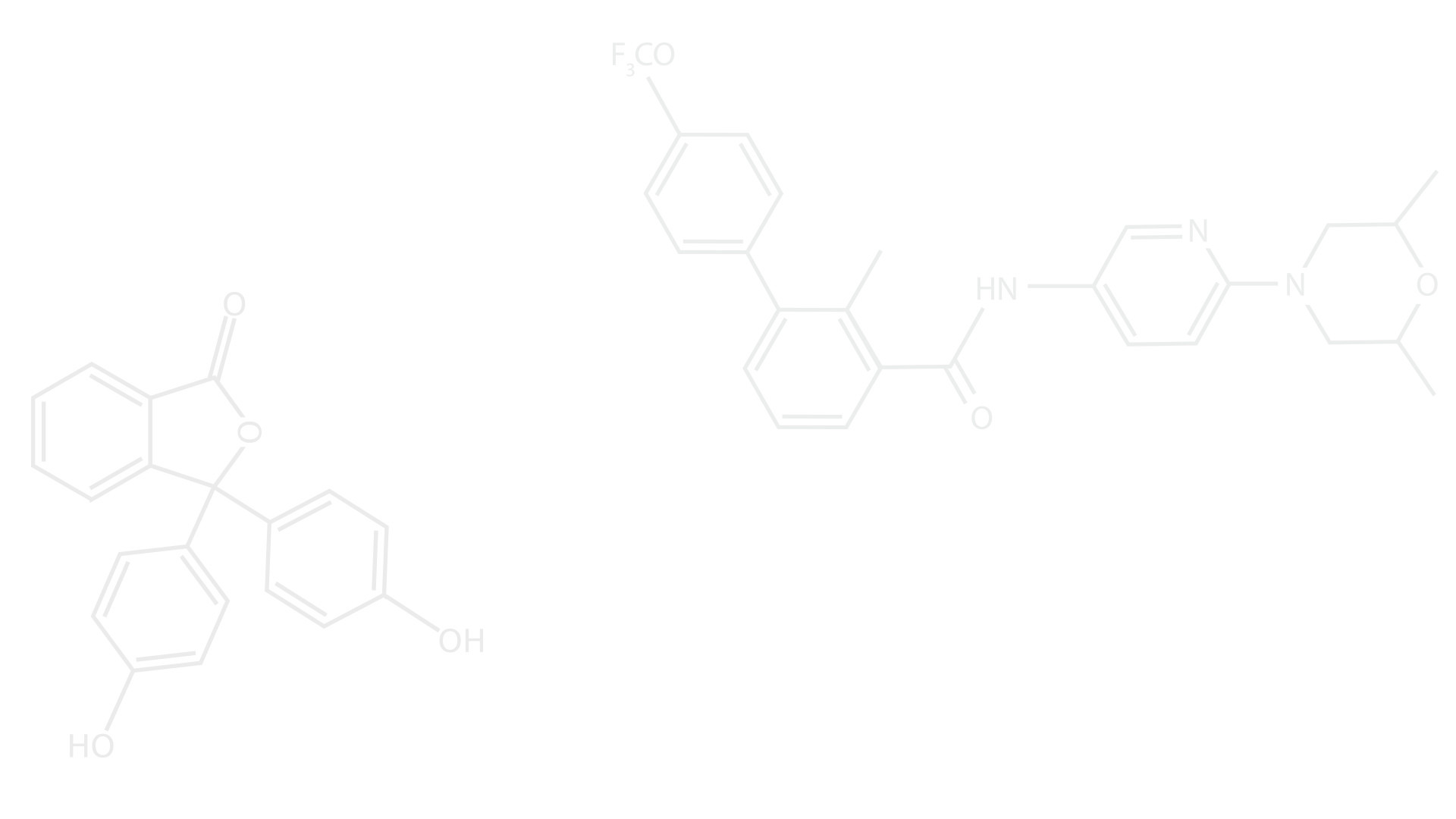
RESEARCH IN THE BEE LAB
Our efforts are aimed at exploiting the huge potential of micro and nanoelectronic systems for diagnostic applications in the agro-food chain as well as fundamental research in health-nutrition relationship.
We focus on the study and development of:
-
Carbon nanotube field-effect transistors
-
Electrochemical biosensors
-
Electrochemical imaging
-
Nanobiotechnology
-
BioMEMS
​​​
Study host-bacterial interactions by a real-time 3D mapping with electrochemical imaging
-
A real-time study of the effect of dietary compounds and host metabolites on gut microbiome
-
Study the effect of herbal extracts on gut epithelial differentiation with electrochemical imaging
Detection of protein and genetic biomarkers using electrochemical biosensors​
-
Detection of phytopathogenic genetic and protein biomarkers
-
Detection of food pathogenic biomarkers
Develop new micro- and nanoelectronic biosensors based on FET (Field-effect Transistor) devices using carbon nanotubes​
-
Develop bioelectronic nanoscale devices for VOC detection
-
Investigation of biomolecular interaction kinetics and structural dynamics
Cell mechanobiology​
-
Study of cell elasticity as a pathological marker using unique MEMS devices​
Nanobiotechnology​
-
Generation of nanohybrids composed of biomolecules (e.g., enzymes) and metals ​
Electrochemical modulation of conformational behavior and biomolecular interactions
-
Characterization of enzymatic activities and binding affinities at bio-functionalized conductive surfaces​
Bioelectronic devices
All-electronic platforms transduce biomolecular interactions, such as binding or conformational changes directly to electrons, resulting in high signal levels (and high sensitivities).
Such devices can be miniaturized to the nanoscale and their potential as biosensors or as tools to study structural dynamics is huge.
A field-effect transistor configuration (FET) is very attractive for biosensing applications and the use of carbon nanotubes (CNT) as channel materials is particularly promising.
Bioelectronic devices in the form of CNT-FETs were demonstrated, for example, in a single-molecule kinetic study of DNA hybridization. Kinetics were strongly affected by electrical potential allowing for electrostatic modulation and enabling the detection of single base mismatches by simply applying potential.
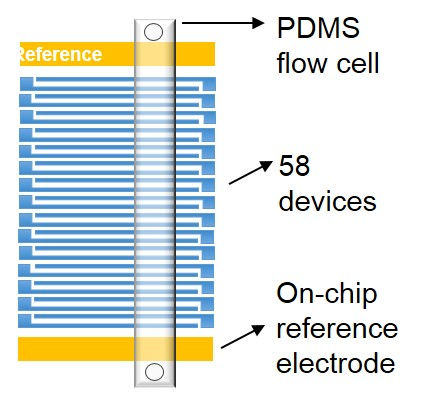
The electrode configuration of an experimental chip. Multiple source and drain electrode pairs and additional reference... electrodes (gates). A PDMS flow cell is stamped over isolated CNT devices.

A simplified circuit scheme showing drain and source potentials, a CNT transistor channel and electrolytic gate applied by an on-chip reference electrode... Different bias schemes are allowed for each device.

Microscope image showing multiple FET devices fabricated on a single chip. Each device consists of a Titanium source and drain... electrodes bridged by a single carbon nanotube.
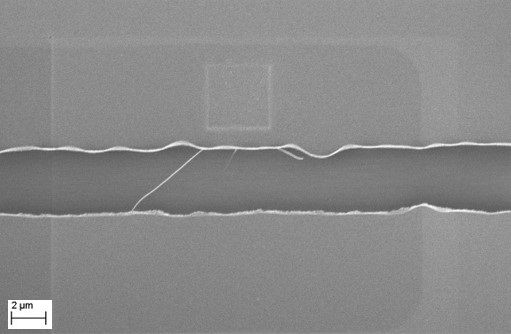
SEM image showing a single CNT forming the conducting channel between source and drain electrodes.
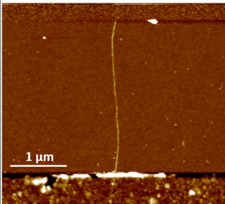
AFM image showing an isolated single-walled CNT bridging source and drain electrodes.
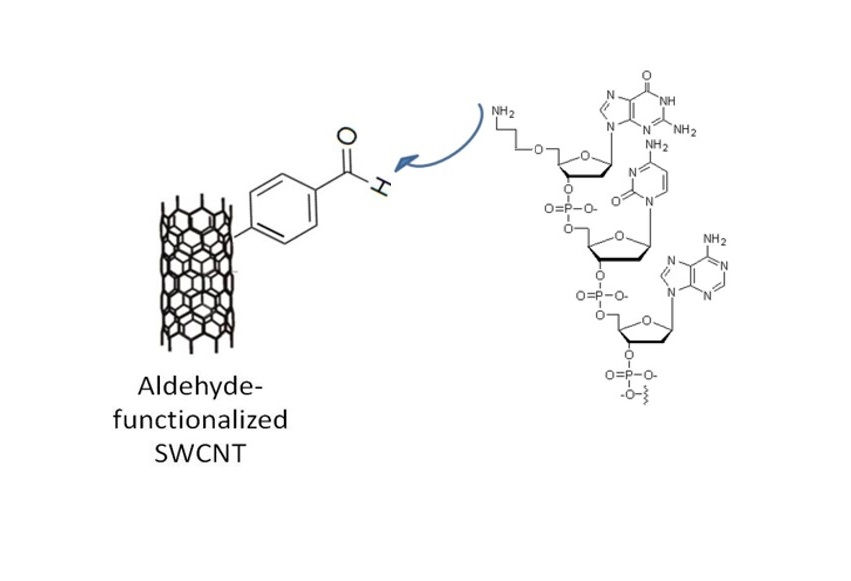
Carbon nanotubes can be covalently functionalized using diazonium chemistry and subsequent tethering of a biomolecule (e.g., ssDNA).
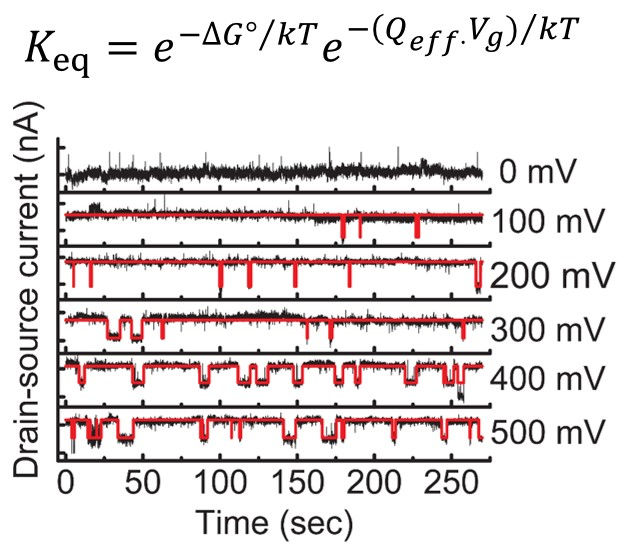
Single-molecule conductance versus time trajectories reflect the hybridization and melting kinetics of a single DNA molecule at the melting temperature under various voltage biases.

The melting rate constant of a complementary DNA target increases exponentially with bias. A target containing a single-base mismatch has a higher melting rate constant at each bias point.

The 'melting potential' (the potential at which Keq=1) is higher for a complementary DNA target than a SNP-containing target.
Electrochemical biosensors
Electrochemical biosensors play a vital role in modern bioanalytic assays with notable applications in the diagnostics arena. These biosensors are devices with user-friendly operation and high-throughput, perfectly suited for the current trend of rapid, on-site diagnostics. Their applications range from biomedical and clinical to food industry, agriculture and environment. We have previously developed, for example, a bioelectrochemical platform that simultaneously detected multiple biomarkers from a tissue sample (biopsy) and a cell sample. Enzymatic activity of an intestinal epithelial differentiation marker was detected directly from biopsies. The same biochip was utilized in an electrochemical immunoassay to map a unique expression pattern of multiple secreted cancer biomarkers and an additional over expressed receptor from a cell culture sample.
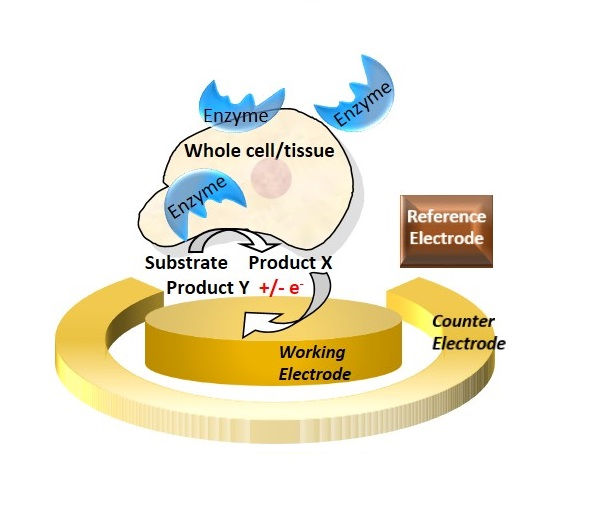
A simple amperometric detection of an enzyme biomarker was demonstrated by adding an electro-active enzyme.. substrate and recording the resulted current signals in an electrochemical cell.
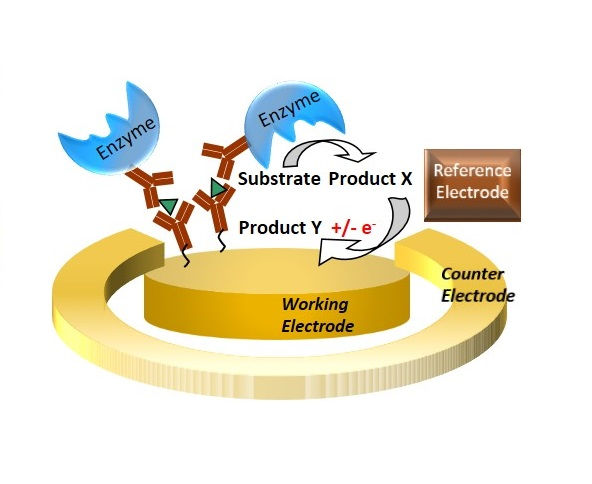
Electrochemical biosensors can employ a method very similar to ELISA. The resulting signal levels and sensitivities are potentially higher.

One of the key elements in biosensors is the functionalization of the transducer with a bio-recognition element. Here, for example, a fluorescent microscopy image of immobilized labeled antibodies to an electrode surface is shown.
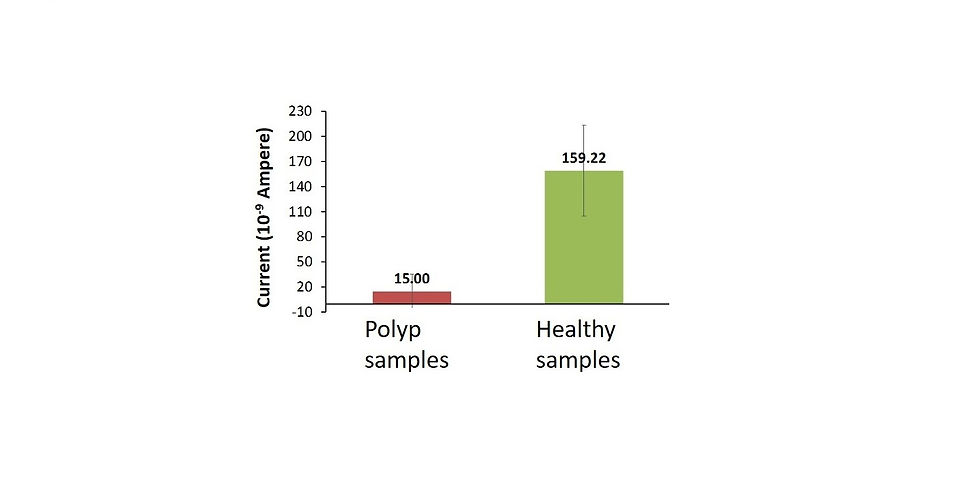
Electrochemical detection of enzymatic activity provided a distinction between tumor (colon cancer) and healthy tissue within 5 minutes in a clinical trial.
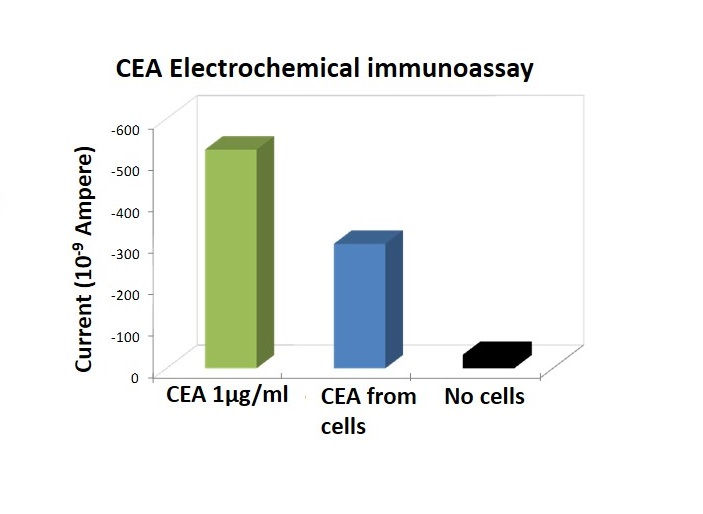
Sensitive detection of a colon cancer biomarker (CEA) from a human cell line using an electrochemical immunoassay. A panel of biomarkers was simultaneously detected.
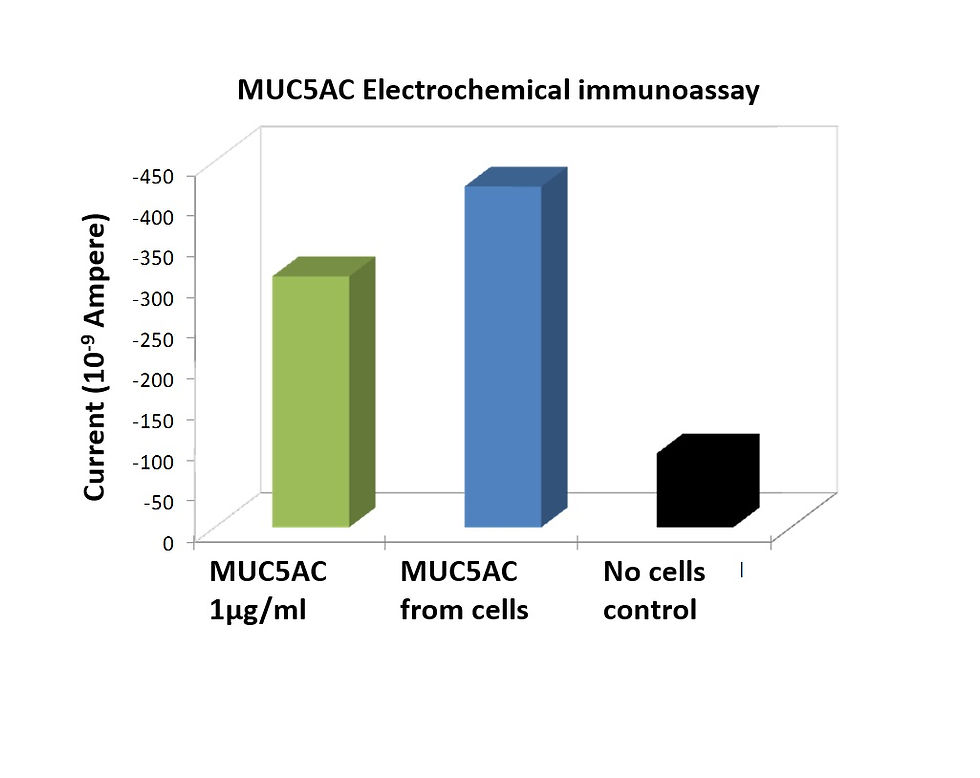
Sensitive detection of a colon cancer biomarker (MUC5AC) from a human cell line using an electrochemical immunoassay. A panel of biomarkers was simultaneously detected.

Illustration of a biochip containing an electrochemical cell with modified electrodes, electrical contacts and a sample chamber. Biochips are inserted into a special 'cartridge' .

An illustration of a platform comprising a multi-potentiostat, a microfluidic system and a sample holder used for mounting a biochip cartridge.

A multi-target analysis can be applied by a biosensor comprising a combination of different bio-electrochemical assays. A panel of biomarkers may be detected yielding an expression map, which increases the diagnostic accuracy significantly.
Nanobiotechnology
The production of nanohybrids comprising active biomolecules and electrically conductive materials (metals or carbon based), results in complex hybrids demonstrating novel properties. These hybrids can be integrated in devices providing them with the desired functionality. For example, effective wiring of enzyme-metal hybrids to electrodes was attained by coating a soluble oxidase with different metals using electroless deposition method. This form of directed metallization is unique since it retains enzymatic activity. Functional hybrids can also be generated by targeting biomolecules to CNT sidewalls using diazonium coupling.
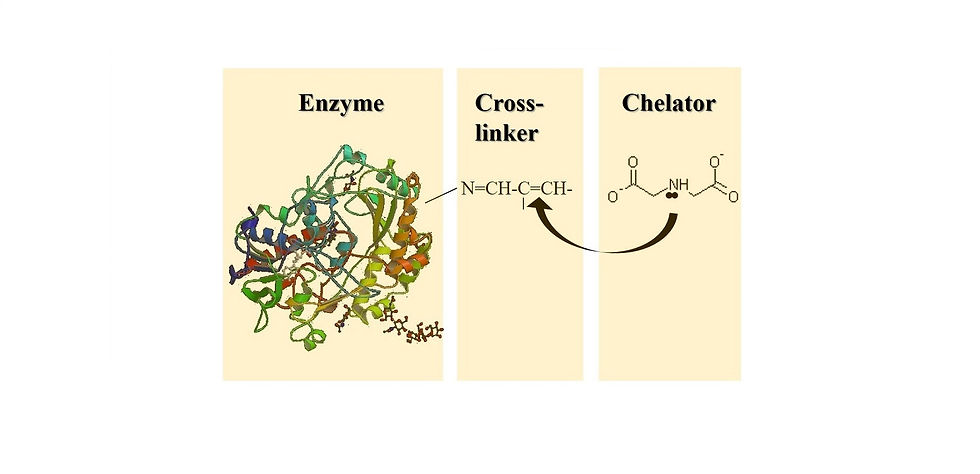
The developed methodology for directed metallization of a single, soluble and active enzyme molecule via electroless deposition.
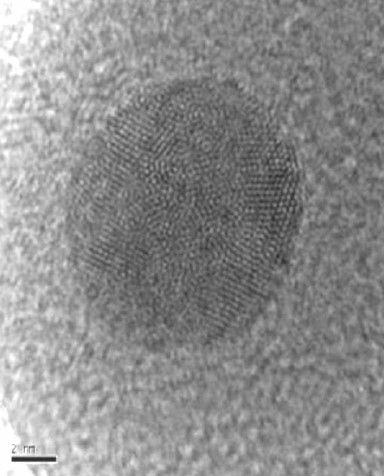
HRTEM micrograph of Glucose oxidase coated with ultra-thin layer of Palladium obtained by electroless deposition of a chemically modified enzyme.

EDS spectrum of a Palladium-gluocose oxidase hybrid verifying the presence of Carbon, Oxygen and Palladium. (Cu originated from the TEM grid).
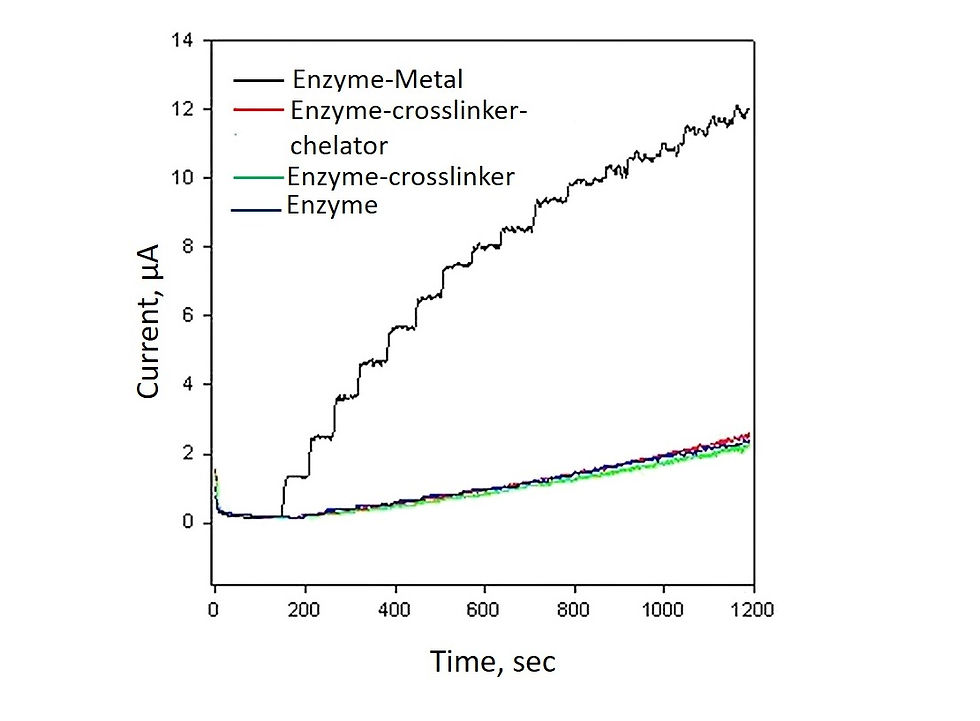
A chronoamperomegram showing the current response of a metal-GOx hybrid to glucose addition. The electrochemical activity is enhanced owing to improved conductivity conferred by the metallic coverage.
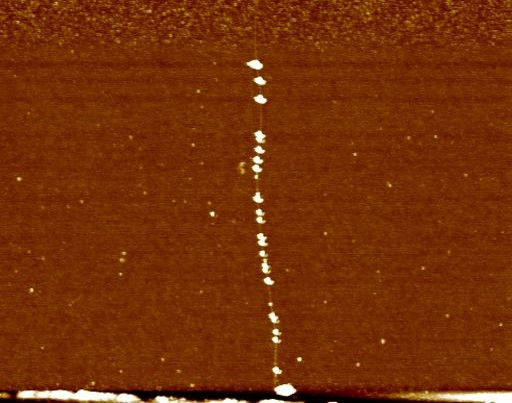
AFM image of an isolated carbon nanotube decorated with gold nanoparticles, which are subsequently used for biomolecule conjugation.
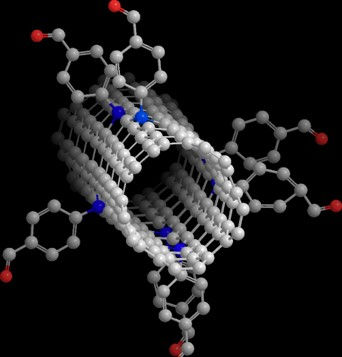
illustration of a single-walled carbon nanotube modified with a diazonium reagent containing an aldehyde group. This functional group is later used for biomolecule conjugation.
BioMEMS
Cells generate, sense, resist and respond to mechanical forces. Differences in mechanical properties of cells correlate with pathophysiological states in human diseases. On-chip single cell analysis offers accurate representation of cell variations. MEMS-based actutaors are particularly promising in this field. MEMS technology can integrate both microsensors and microactuators in a single chip used for biological applications. An electrostatic MEMS chip, the 'Bio-RAM', was applied in the mechanical characterization of cancer cell elasticity. The device integrated a microfluidic channel with a special 'comb-drive' configured actoator allowing for a single cell capture and analysis.

A schematic description of the 'BioRAM' - electrostatic comb-drive actuator used for force measurements of single cells. Once voltage is applied the electrostatic force drives the 'Ram' towards the captured cell. The extent of applied voltage required for cell deformation is recorded and analysed as well as the time for cell recovery.
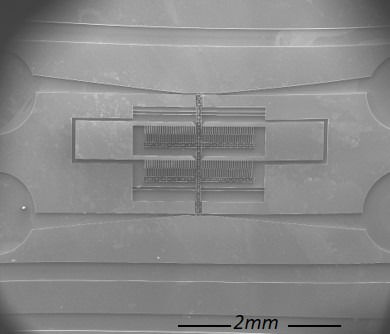
ESEM image of the Bio-RAM device including the four reservoirs (1 mm "wells") and microfluidic channels enabling simple cell injection. The comb-drive and backbone of the device are also shown.
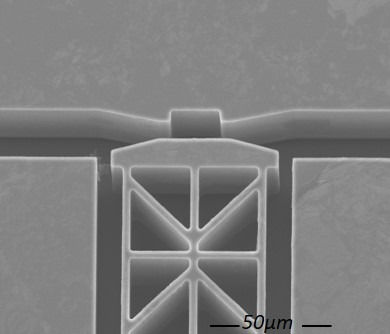
SEM image showing the moving tip ('ram') of the device and the chamber designed for single cell capture and force measurements.
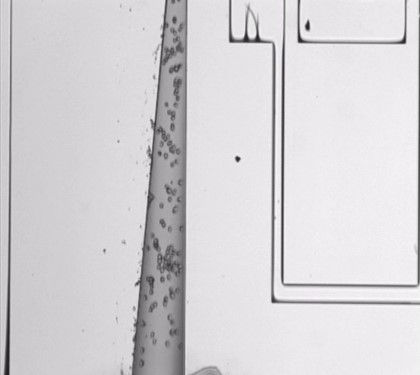
Upon injection, cells rapidly flow through the microfluidic channel, which narrows until reaching the single cell chamber.

A single cell is 'clamped' by the actuated tip (ram) at its apical side. Cell is compressed against the cell chamber while voltage is applied.

The same cell from the previous image is being continuously compressed and released. Severe deformation is evident until a 'sickle-like' morphology is seen. The cell, nevertheless, retains its integrity.